Accepted 30 November 2020
Available Online 14 December 2020
- DOI
- https://doi.org/10.2991/mathi.k.201206.001
- Keywords
- Melatonin
inkjet printing
mucosal pellicle
TR146 cells
drug delivery - Abstract
There is a growing interest in the use of melatonin in preventing radiation-induced mucositis with potential beneficial effects including prevention of mucosal damage, the emergence of ulcers and the loss of proliferative progenitor stem cells caused by radiation. Local drug delivery to the oral cavity is influenced by the oral mucosal pellicle which serves as a protective barrier between the oral epithelial surface and the external environment. In this study, we explore two key areas. Firstly, the use of thermal inkjet printing to improve the precision of drug deposition on polymeric oral films. Secondly, investigate melatonin release and penetration across an ex vivo oral mucosal pellicle model into the epithelial cancer cell line (TR146 cells). Our findings show that the deposited melatonin crystallized on the surface of the Polyvinyl Alcohol (PVA) film as the jetted droplets dried, consistent with previous work on in-jetted pharmaceutical solutions. The solid state of the deposited melatonin was further confirmed in attenuated total reflection Fourier transform infrared studies as crystalline. In addition, no new bonds detected which indicates the absence of new chemical bonds and interactions between melatonin and PVA. Investigating melatonin release and penetration across ex vivo oral mucosal pellicle model into the epithelial cancer cell line (TR146 cells) found that the amount of melatonin released was lower in the presence of saliva, likely due to the saliva initiating the formation of the mucosal pellicle through mucin–mucin interactions.
- Copyright
- © 2020 The Authors. Published by Atlantis Press B.V.
- Open Access
- This is an open access article distributed under the CC BY-NC 4.0 license (http://creativecommons.org/licenses/by-nc/4.0/).
1. INTRODUCTION
Mucositis (defined as inflammation and/or ulceration of the mucosa) can affect the entire alimentary track and is a common and severe toxic side effect of chemo-radiotherapy, commonly experienced by patients with head and neck cancers. The oral cavity is the most affected site due to its increased sensitivity and vulnerability to chemo-radiotherapy compared with other parts of the body. Xerostomia (dry mouth) is also commonly associated with oral mucositis due to decreased salivary flow post chemo-radiotherapy. Mucositis strongly influences patient adherence to cancer therapy treatment protocols, as if not tolerated it can result in the need for dose reductions, treatment delays or discontinuation, therefore affecting survival outcomes and in turn directly impacting cancer survival rates [1].
Five overlapping stages are thought to be involved in mucosal injury: initiation, upregulation, message generation, ulceration and healing. Mucositis starts when mucosa is exposed to reactive oxygen species from cytotoxic agents, resulting in direct damage to cells and triggering a cascade of inflammatory reactions. This activates the nuclear factor-kappa B innate immune pathway which leads to local increases of pro-inflammatory cytokines including tumor necrosis factor and interleukin-6 [2]. The positive feedback loops amplify and accelerate the whole inflammatory process which eventually leads to ulceration. The last stage is the healing process where the growth of epithelial cells is downregulated, and differentiation starts to facilitate wound healing. The symptoms begin to subside during the healing stage and healing is usually completed a month after the last dose of radiotherapy [3].
A number of preventative and therapeutic approaches have been used to manage oral mucositis, such as antimicrobial mouthwashes, glutamine and prostaglandin E1 analogs [4] and anti-inflammatory agents [5]. However, there is no unequivocal evidence to suggest that these agents are effective in treating or preventing oral mucositis.
There is a growing interest in the use of melatonin in preventing radiation-induced mucositis. Two enzymes (i.e. aralkylamine N-acetyltransferase and acetylserotonin O-methyltransferase) involved in melatonin synthesis are expressed in oral mucosa and also in salivary glands [6]. Melatonin is also found in saliva and is thought to passively enter the mucous through the circulatory system in salivary glands (parotid, submaxillary, and sublingual glands) [7]. Other work has suggested that melatonin could play a valuable role in preventing mucositis with beneficial effects including prevention of mucosal damage, the emergence of ulcers and the loss of proliferative progenitor stem cells caused by radiation [8].
As a site for drug delivery, the oral cavity offers various advantages compared with the conventional oral route. These include avoidance of first-pass hepatic metabolism, provision of a large area and relatively immobile surface which is suitable for controlled and localized drug delivery with avoidance of systemic effects and most importantly high patient acceptability, which can in turn improve patient compliance. When considering drug delivery to the oral cavity especially for the local treatment of oral mucositis, a factor that needs to be considered is the oral mucosal pellicle. The oral mucosal pellicle serves as a protective barrier between the oral epithelial surface and the external environment, but also acts as a barrier to drug delivery. Previously, we identified [9] MUC5B and MUC7 to be strongly retained on the buccal cell surfaces forming a thin layer of the pellicle [10] along with secretory immunoglobulin A (IgA) have a role in initiating the formation of the mucosal pellicle through mucin–mucin interactions with membrane-bound mucins on cells [11]. There is a need for further work to understand the impact the oral mucosal pellicle has on the efficacy of a drug delivery system in the healthy and diseased state.
Polymeric oral films are a well-established solid dosage form to administer drugs to the oral cavity. Ideal characteristics of polymeric oral films include sufficient loading capacity, uniform drug distribution, flexible, non-toxic, biocompatible and biodegradable [12]. The main advantage of polymeric oral films is their high patient acceptability and compliance making them a very promising dosage form for oral mucositis treatment. A major disadvantage of these systems is the ability to achieve uniform drug distribution throughout the oral film. Most strategies have explored the incorporation of the drug into the polymeric matrix prior to formulation in the case with solvent casting systems [13]. An alternative approach is to cast the film separately and then deposit the API onto it using techniques such as flexography [14], thermal inkjet printing [15] and a combination of the two [16].
In this study, we explore two key areas. Firstly, the use of thermal inkjet printing to improve the precision of drug deposition on polymeric oral films. Thermal inkjet printing has the advantage of being able to deposit very small volumes (5–15 pL/droplet) with high precision [15] and has successfully been applied to various pharmaceutical applications, especially in manufacturing drug products [17,18]. Secondly, investigate melatonin release and penetration across an ex vivo oral mucosal pellicle model into the epithelial cancer cell line (TR146 cells).
2. MATERIALS AND METHODS
2.1. Materials
Melatonin powder, 99+% (lot: Q20D022) was purchased from Alfa Aesar, USA. Absolute ethanol (lot: 1730528) was purchased from Fisher Scientific, UK. Polyvinyl alcohol, 99+% hydrolyzed (lot: MKBX9179V), Dulbecco’s Phosphate Buffered Saline (DPBS) (lot: RNBF9336) and Radioimmunoprecipitation Assay (RIPA) buffer (lot: SLBK8528V) were purchased from Sigma-Aldrich, UK. PBS, advanced Dulbecco’s Modified Eagles Medium (DMEM)/F12 (lot: 1669928), 0.25% trypsin-Ethylenediaminetetraacetic acid (EDTA) (lot: 1726653) and 0.4% trypan blue stain (lot: 1705607) were purchased from Gibco, UK. TR146 cell lines were grown and provided by King’s College London Dental Institute Salivary Research Unit, UK.
2.2. Methods
2.2.1. Pharmaceutical film preparation using solvent casting method
Polyvinyl Alcohol (PVA) granules were dissolved in distilled water at 100°C to produce a 10% w/v PVA polymer solution. The temperature was maintained at 100°C [with magnetic stirring at a rotation speed of 400 Rotation per Minute (rpm)] until all granules were added to the distilled water. The temperature was then reduced to 60°C for a further 10 min and the solution was left stirring until all the granules had completely dissolved. The polymer solution was transferred into petri dishes and dried for 24 h.
2.2.2. Thermal inkjet printing of melatonin
Melatonin was dissolved in ethanol to produce a concentration of 50 mg/mL. 1 mL of solution was then loaded separately into two sterilized black ink cartridges for printing. This solution were then printed onto PVA films using a modified Hewlett-Packard (HP) 5940 Deskjet (USA). The modifications to the printer have been previously reported [19,20] Melatonin solution was printed in multiple passes to deposit 10 layers of melatonin onto PVA films. Printing was conducted in triplicate. The printing template used was a set of 1 × 1 cm2.
2.2.3. Scanning electron microscopy
The surface morphology of PVA films and the printed PVA films containing melatonin were visualized using a Scanning Electron Microscopy (SEM) (Hitachi S4000, UK) at an accelerating voltage of 6 kV. The samples were coated with gold ions under vacuum using a gold sputtering device (Quorum Q150T S, UK) and were mounted on the aluminum stabs with double-sided adhesive carbon tape. The samples were then scanned at a suitable magnification and the micrographs were recorded to study the surface morphology.
2.2.4. Attenuated total reflection Fourier transform infrared spectroscopy
Attenuated Total Reflection Fourier Transform Infrared (ATR-FTIR) spectra were obtained using a Perkin–Elmer Frontier ATR-FTIR spectrometer to assess the compound functional groups and identify any possible interactions between melatonin and PVA. The spectra of melatonin, PVA granules, PVA film and the deposited PVA film formulations which consisted 10 printed layers of melatonin were recorded in the frequency range of 4000–600/cm with a resolution of 2/cm and four times scanning. Each sample was recorded in triplicate.
2.2.5. UV quantification of melatonin deposited onto PVA films
Imprinted melatonin templates were dissolved in 10 mL of ethanol until all the contents were dissolved. Solutions were then analyzed spectrophotometrically at 278 nm to assess the amounts of melatonin in each sample.
2.2.6. Preparation of cell homogenates for quantification
TR146 cells were grown in medium to desired level of confluency in a T75 flask. The medium was aspirated, and the cells were washed with 5 mL of DPBS and the DPBS was then aspirated. 3 mL of Trypsin solution was added to the T75 flask and the flask was then placed into a CO2 incubator (Heraeus Heracell) at 37°C for about 10 min. After 10 min, 3 mL of DMEM was added to the flask to neutralize the trypsin. The solution with the cells was then transferred to a sterile 15 mL conical centrifuge tube and pelleted by centrifugation at 1500 rpm for 5 min by using a centrifuge (Heraeus Fresco 21) and the supernatant was aspirated. DMEM (4 mL) was added to the conical tube to wash the cells and resuspend the pellets. 100 µL of cell suspension was transferred into a bijou container and 400 µL of trypan blue stain was added to the suspension and mixed gently. 100 µL of trypan blue-treated cell suspension was transferred to both the chambers underneath the coverslip of a haemocytometer and were visualized using a light microscope to count the live (1 × 105), unstained cells to enumerate the cell density. 1 mL of cell suspension with the cell density of 1 × 105 cells was transferred into the wells of sterile 12-well cell culture plate and the cells were incubated for 24 h at 37°C.
2.2.7. Saliva collection and cell incubation
Unstimulated whole mouth healthy human saliva was used as a mucin source to form a layer of adsorbed salivary proteins, known as the oral mucosal pellicle, which is bound to the surface of epithelial cells. Unstimulated whole mouth human saliva was collected and centrifuged at 13,500 g for 5 min. The supernatant was mixed with DMEM medium to create a 1:1 concentration. 1 mL of the mixture was added to the cells in triplicate that requiring mucosal pellicle for testing and 1 mL of DMEM was added to the cells that did not require salivary pellicle for 20 min incubation at 37°C. Test samples, i.e. PVA film formulations that contain 10 printed layers of melatonin were placed above the TR146 cells and 1 mL of the DMEM media were then added on top of the test samples. The well plates were left inside the CO2 incubator for 24 h incubation at 37°C.
2.2.8. Cell homogenization and ultrafiltration
The TR146 cells were washed with 1 mL of ice cold DPBS and then aspirated. 50 µL of ice cold RIPA buffer was added to each well and the cells were removed from the base of the wells by using a cell scrapper. The RIPA buffer solutions were then transferred into 1.5 mL microcentrifuge tubes and vortexed thoroughly for 30 s, transferred into an ice box and placed on an agitator for 15 min. The tubes were then centrifuged at 13,500 g for 5 min at 4°C. The pellets were discarded, and the cell homogenates were transferred into 10 kDa molecular weight cut off concentrators (Vivaspins, Sartorius) and then centrifuged at 4000 g for 30 min at 4°C. The recovered samples were then transferred into 0.5 mL tubes and stored in a freezer at −20°C until analysis.
2.2.9. Quantitative determination of melatonin released into TR146 cells
One hundred microliter of each diluted sample solution was transferred to a 96-well plate and the solutions were analyzed using a UV microplate reader (SpectraMax 190) at 278 nm to assess the amounts of melatonin in each sample. Each sample was measured in duplicate.
2.2.10. Statistical analysis
Data were assessed for normality and if suitable ANOVA followed by student’s t-test were conducted to determine the statistically significant difference between the amounts of the melatonin released into the cells in two different samples.
3. RESULTS
3.1. Scanning Electron Microscopy Analysis
Figure 1 shows deposited melatonin on PVA pharmaceutical films printed in 10 layers under the print head on a 1 cm2 area. The use of different number of printing layers was to observe any trends in drug amount deposited. UV quantification showed that printing 1 × 1 cm2 of melatonin solution in multiple scans and passing the films under the print head 10 times deposited 350.68 ± 0.040 µg of melatonin. The volume of the ink solution to be jetted during printing varies for all solutions and is dependent upon a number of physical properties including viscosity, density, surface tension and concentration of printing solutions [21]. These parameters can therefore influence the droplet formation mechanism and subsequent drop size at a given voltage [22].
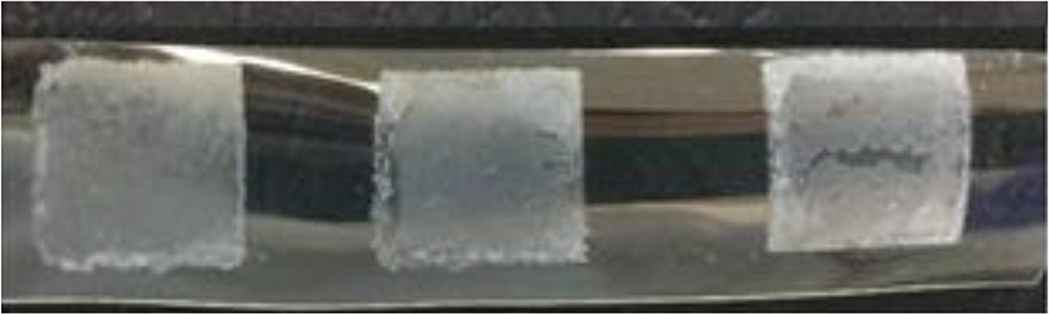
Deposition of melatonin onto PVA films 10 times under the print head for the set of 1 × 1 cm2.
An SEM micrograph of the surface of the PVA film is illustrated in Figure 2A and the image shows the surface was smooth and homogenous. The SEM images of the PVA films deposited with layers of melatonin is represented in Figure 2B. Figure 2B shows that the deposited melatonin exists in a crystalline structure with irregular shapes, indicating that melatonin crystallized on the surface of the polymer film as the jetted droplets dried, consistent with previous work on in-jetted pharmaceutical solutions [15,23].
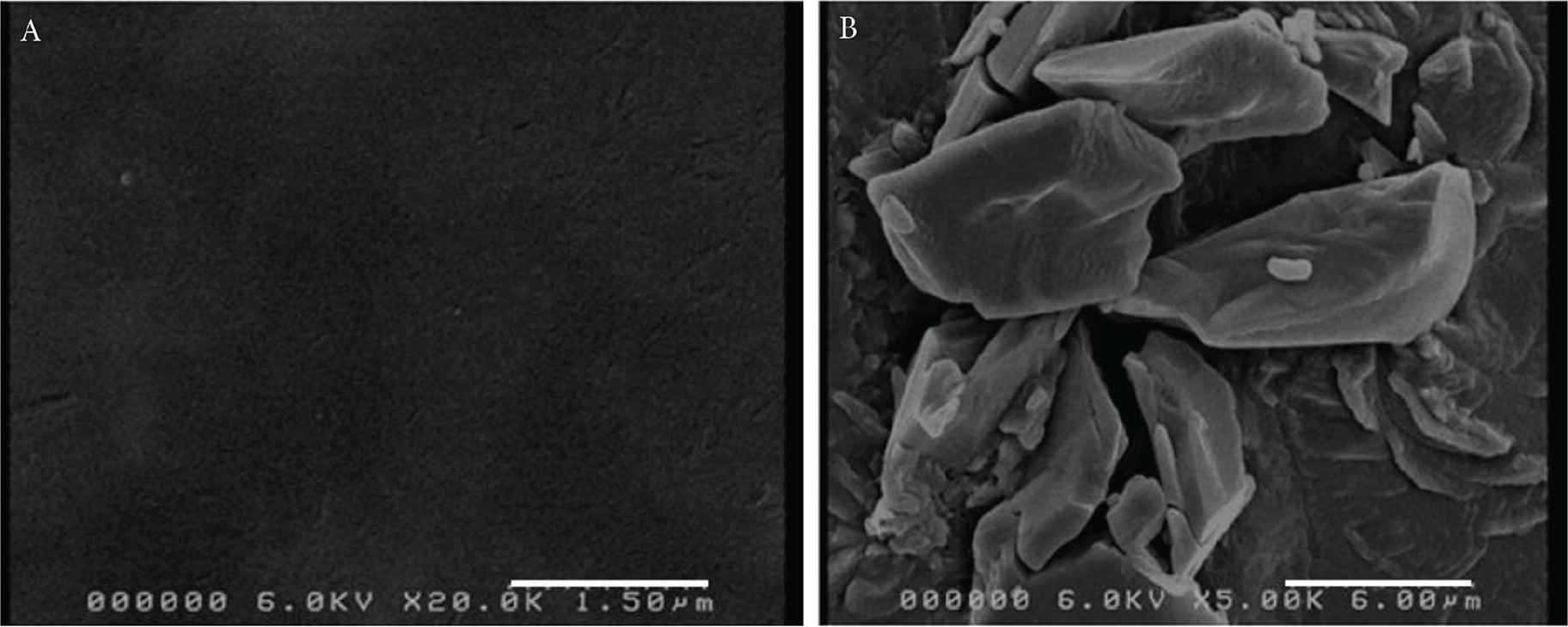
SEM images of (A) PVA film and (B) PVA film formulations with 10 printed layers of deposited melatonin.
3.2. Attenuated Total Reflection Fourier Transform Infrared Analysis
Scanning electron microscopy studies suggested that deposited melatonin was present on the PVA oral films in its crystalline form. However, the true solid state of the deposited melatonin was not known. ATR-FTIR studies investigated the solid state of the deposited melatonin as well as identifying any potential interaction between PVA and deposited melatonin (Figure 3A–3D). ATR-FTIR spectra of melatonin deposited film formulations showed characteristic absorption melatonin peaks (Figure 3B) and no new bonds detected which indicates the absence of new chemical bonds and interactions between the deposited melatonin and PVA film.
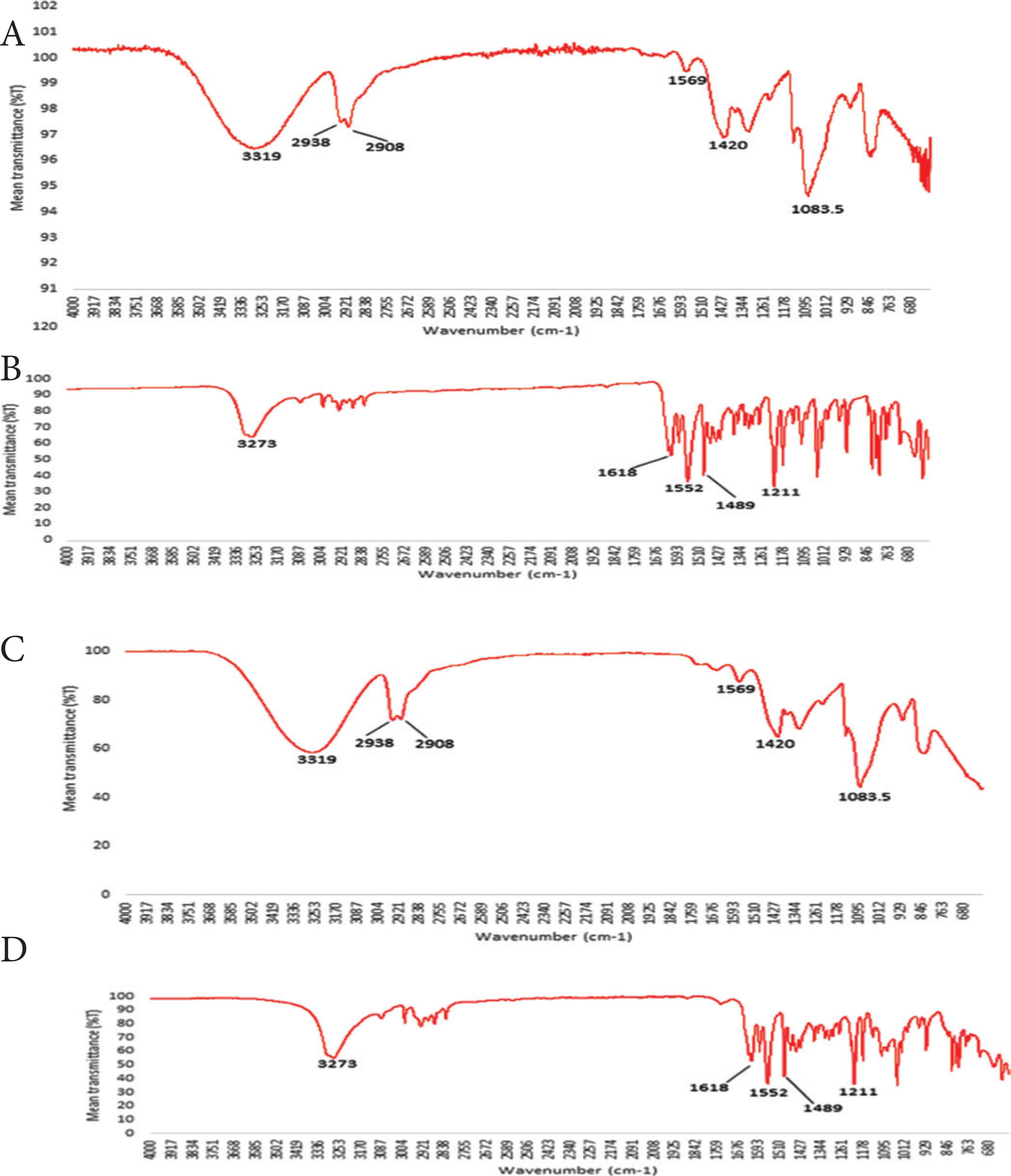
ATR-FTIR spectra of (A) PVA granules, (B) raw material melatonin, (C) PVA film and (D) PVA film formulations with deposited melatonin.
3.3. Quantitative Determination of Melatonin Released into TR146 Cells
The oral cavity is constantly washed by saliva (approx. 0.5 mL/min in healthy adults), diluting any drug present. This therefore can reduce the contact of a topically applied formulation as well as its bioavailability. The oral mucosa is lined with a layer of mucus formed from salivary mucins Muc5b and Muc7 binding to cell membrane bound mucins [10]. In this study, we replicated the oral mucosal pellicle by adding saliva to the TR146 cells as previously described [11] to determine the effect of the pellicle on the amount of melatonin transferred into the TR146 cells.
The calculated amount of deposited melatonin (10 layers) taken up by TR146 cells (in the presence and absence of whole mouth saliva) after 24 h of incubation was evaluated. The amount of melatonin taken up by the TR146 cells was similar (p = 0.27) when tested with saliva (55.52 ± 1.80 µg) and without saliva (59.02 ± 2.72 µg). These findings confirm that melatonin release was not influenced by the presence of saliva.
4. DISCUSSION
In this study, we explore two key areas. Firstly, the use of thermal inkjet printing to improve the precision of drug deposition on polymeric oral films. Secondly, investigate melatonin release and penetration across an ex vivo oral mucosal pellicle model into the epithelial cancer cell line (TR146 cells).
Polymeric oral films were made using PVA. PVA is a commonly used synthetic water soluble polymer with good mucoadhesive properties to make pharmaceutical films due to its versatile properties and good film-forming ability [24,25]. Mucoadhesive polymers are an essential tools of choice in formulating remedies for dry mouth treatment [26]. Saliva substitutes that contain mucoadhesive polymers can mimic the salivary effect in the oral cavity and can re-saturate the impaired mucus layer in dry mouth.
Deposited melatonin on PVA films was present in its crystalline form. This could prove advantageous in terms of drug stability. As no interaction between PVA and deposited melatonin was observed in ATR-FTIR studies, it is thought that the melatonin crystals lie on top of the PVA film but are not chemically bound to the PVA film.
Ex vivo drug release studies using an oral mucosal pellicle model into TR146 cells saw that the amount of melatonin released was lower in the presence of saliva. This is likely due to the saliva initiating the formation of the mucosal pellicle through mucin–mucin interactions. This mucosal pellicle therefore act as a barrier for drug delivery to the target cells resulting in a lower drug uptake. In the presence of saliva, the printed melatonin must cross the mucosal pellicle to reach the target, TR146 cells. These findings are in support with reports that the salivary pellicle can serve as a barrier to drug delivery therefore, affecting the rate of buccal absorption.
5. CONCLUSION
We successfully deposited melatonin on to PVA pharmaceutical films using inkjet printing and observed its uptake in an ex vivo oral mucosal pellicle model. Quantitative determination of the amount of melatonin released into TR146 buccal mucosa cells did not see any significant differences in the amount of melatonin released into the cells in the presence and absence of saliva.
CONFLICTS OF INTEREST
The authors declare they have no conflicts of interest.
FUNDING
This research received no external funding.
ACKNOWLEDGMENTS
The authors would like to thank William Bill Luckhurst (Department of Physics, King’s College London) and Ben Blackburn (Department of Physics, King’s College London) for their assistance with the scanning electron microscopy studies using the Hitachi, S4000.
Footnotes
REFERENCES
Cite This Article
TY - JOUR AU - Yin Ming Khor AU - Simon Gaisford AU - Guy Howard Carpenter AU - Bahijja Tolulope Raimi-Abraham PY - 2020 DA - 2020/12/14 TI - Inkjet Printed Melatonin on Poly(vinyl alcohol) Oral Films: Uptake in an Ex Vivo Oral Mucosal Pellicle Model JO - Materials Highlights SP - 1 EP - 6 VL - 2 IS - 1-2 SN - 2666-4933 UR - https://doi.org/10.2991/mathi.k.201206.001 DO - https://doi.org/10.2991/mathi.k.201206.001 ID - Khor2020 ER -